Mitochondrial DNA Can Now Be Edited Using This Frankenstein Like Molecular Machine Put Together By Researchers
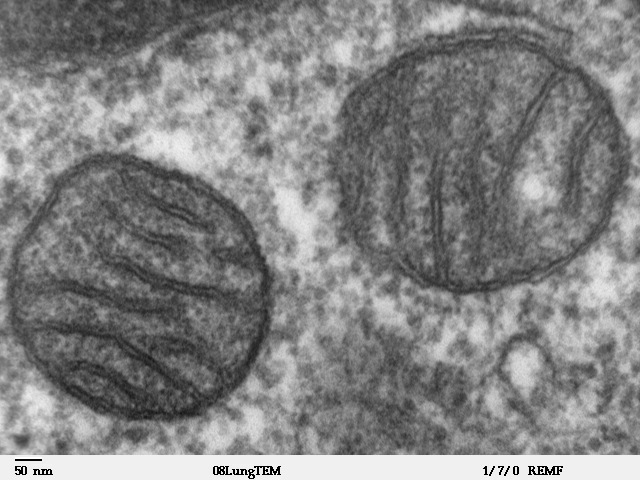
Ever since the discovery of DNA as the molecule that contains genes, genetic engineering has seen steady strides in capability. Today, multiple different artificial protein complexes have been developed, that have made gene editing much easier than before. This post covers one such molecular machine, that has been recently developed to edit genes found in cell organelles called mitochondria, a feat that was pretty difficult until recently. I will describe, step by step, the different molecular parts from disparate proteins, that were stitched together into this complex, and what the relevance of each of these parts is.
DNA in human cells is found in two locations, the nucleus and the mitochondria. You can read more about the structure of DNA in this post of mine. The nucleus has an ideal environment for DNA. The mitochondria, however, are not an ideal environment for DNA to be in. This is because the respiratory reactions of citric acid cycle and electron transport chain that happen there generate certain chemicals called reactive oxygen species (ROS). These ROSs are extremely reactive, leading to them react with DNA and damage it. This is perhaps the reason why over time, a lot of the mitochondrial DNA has gradually shifted to the nucleus.
In fact, this phenomenon of DNA being transferred to nucleus has been observed not just in mitochondria, but in chloroplasts of plant cells as well. Chloroplasts are the organelles in plant cells where photosynthesis takes place, and just like mitochondria, they too contain their own DNA. And just like the respiratory reactions in mitochondria, the photosynthetic reactions in chloroplasts also produce ROSs that damage DNA.
In fact, when researchers recently artificially transferred some chloroplast genes into the nucleus to shield rice plants from climate change, the benefits to the plants were so pronounced that it even increased the yield of rice, which wasn’t even the primary objective of the researchers. You can read more about this study in this post of mine.
Editing mitochondrial genes has always been an area of research, as many genetic diseases have their origin in faulty mitochondrial genes. However, mitochondrial genes cannot be edited using the molecular machinery used to edit nuclear genes, as that machinery is not capable of crossing the outer and inner membranes of mitochondria, and enter its innermost region that contains the mitochondrial genes. Recently, researchers have taken parts of different, unrelated proteins, and joined them together to make a novel protein that can effectively edit mitochondrial genes. They named this protein DddA-derived cytosine base editor (DdCBE).
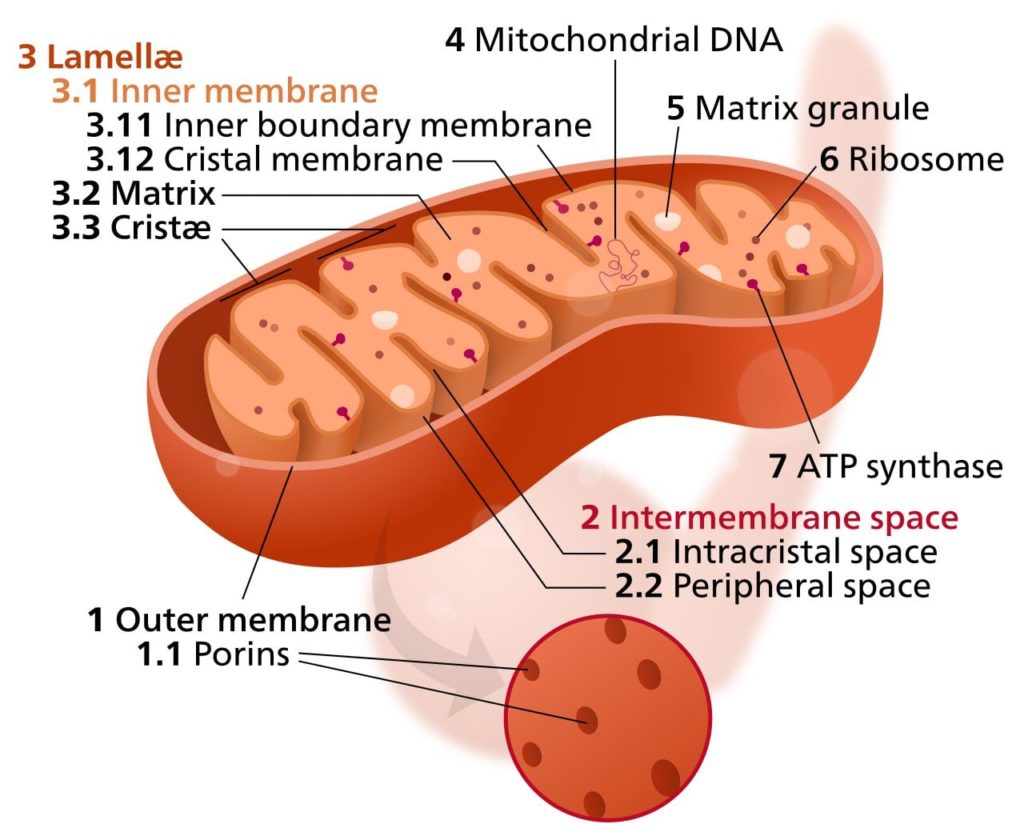
A Bacterial Toxin Harmful To Humans Was Repurposed As A Gene Editor
Genetic information in DNA is coded by combining four types of molecules, namely adenine, thymine, guanine and cytosine in different combinations, just like information in the English language is stored by arranging the 26 letters in various combinations. Central to the effort to edit mitochondrial genes is a recently discovered bacterial toxin called DddA, which converts cytosine into another molecule called uracil. But to use this toxin for editing human mitochondrial DNA, researchers first had to ensure that the toxin doesn’t harm the human patients.
This was done by splitting the toxin into two parts, neither of which can function without the other. These two halves are called split-DddAtox halves. So, even if both these parts are introduced into human cells simultaneously, they fail to harm the cell as they are not united. Next, researchers had to ensure that the two parts of the toxin come together to carry out their function of converting cytosine to uracil, exactly where the researchers need them to, which is the site in the mitochondrial DNA that needs to be edited. For this, they used another protein called TALE.
TALE Proteins
TALE proteins are DNA binding protein molecules that can be engineered to bind specific sites on DNA, by recognizing the specific sequence of A, T, G and C at that site of DNA. This is like asking someone to highlight every phrase in an English book, that contains the word “car”. By engineering the TALE protein to bind the site on mitochondrial DNA that needed to be edited, and then fusing each of the two halves of the DddA toxin to a separate TALE protein molecule, researchers could now direct the two halves of the DddA toxin to the same site of interest in mitochondrial DNA.
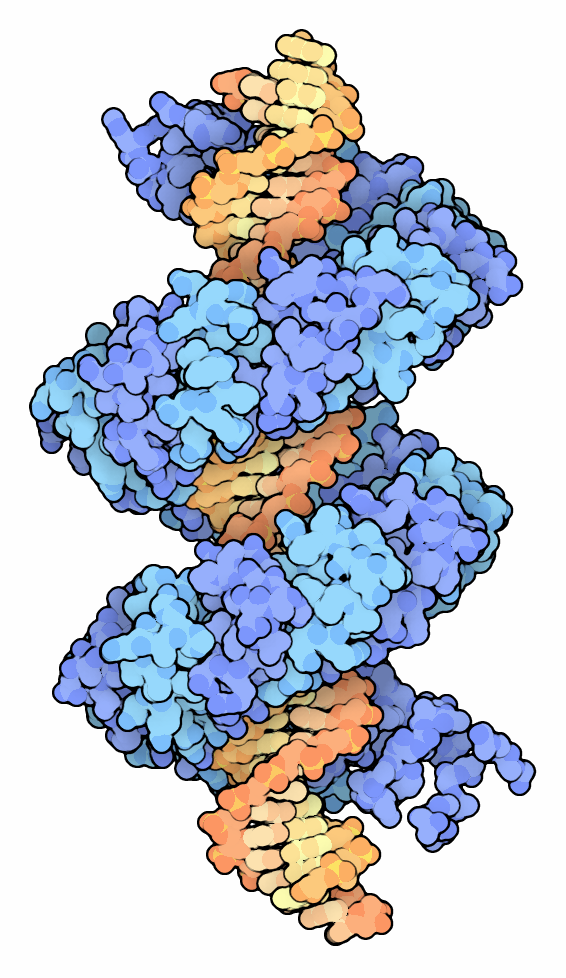
Once the two halves met there, they could together carry out the toxin’s function of converting cytosine to uracil. The next problem that needed to be addressed was how to make the two toxin halves fused to TALE proteins enter the mitochondria in order to access the mitochondrial DNA. This is no easy task, as mitochondria are bound by two concentric membranes, that don’t allow most proteins to pass through.
Mitochondria Targeting Signal Was Added To The Protein Complex To Help It Enter Mitochondria
To help the protein complex cross the two mitochondrial membranes and reach mitochondrial DNA, researchers joined an amino acid sequence (a small protein) to the complex, which is usually a part of those proteins that are naturally transported into mitochondria by the cell’s machinery. This was like someone giving you the password to an account. By doing this, the toxin halves fused to TALE proteins were able to gain entry into mitochondria.
Unlike this new system, the CRISPER-Cas9 (another engineered DNA editing system) system is unable to gain entry into mitochondria. This is likely because the CRISPER-Cas9 system includes not just protein molecules, but RNA molecules as well. And while the mitochondria’s transport machinery allows entry to proteins containing the mitochondria targeting signal, there is probably no machinery in mitochondria to allow entry to RNA molecules.
There was just one last challenge to be addressed. The uracil produced by cytidine deaminase by modifying cytosine, is soon removed by a mitochondrial enzyme called uracil DNA glycosylase. This is followed by re-insertion of a cytosine in its place, which is how the mitochondrial machinery naturally repairs changes in its DNA. It happens because uracil is naturally found in RNA, not DNA.
Attaching Uracil Glycosylase Inhibitor To The System Prevents Mitochondria From Reversing The Edits In Its DNA
To prevent this repair machinery from reversing the edits by removing the uracil, the researchers attached yet another protein, called uracil glycosylase inhibitor (UGI), to the system. As its name suggests, this protein prevents DNA glycosylase from removing the newly created uracil from the mitochondrial DNA site being edited by the construct. It was like turning off the autocorrect in your phone.
The final version of the protein complex therefore, consisted of a mitochondrial targeting signal to help the complex enter the mitochondria, a TALE protein that would bring the complex to the specific site on mitochondrial DNA to be edited, one of the two DddAtox halves, which on joining the other half from another copy of the complex, would edit the DNA by converting cytosine to uracil, and a UGI to prevent the reversing of the edit done on that site. This engineered complex has been named the DdCBE (DddA-derived cytosine base editor).
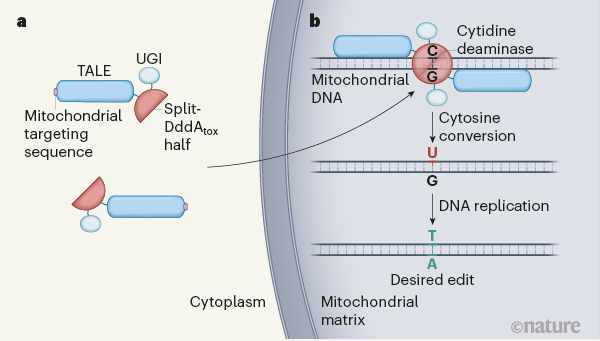
Conclusion
The CRISPR-Cas9 system has made it possible to edit genomic DNA, but the molecular complex is unable to edit mitochondrial DNA because it cannot enter mitochondria. The DdCBE has been designed to be able to enter mitochondria, giving us for the first time, the ability to edit mitochondrial DNA. Some improvements could still be made. For example, in its current form, the DdCBE can edit only those Cytosines that are preceded by a thymine. This limits the sites on mitochondrial DNA that can be edited.
However, this is still a significant step ahead in our efforts to edit faulty mitochondrial DNA, which is the cause of several genetic disorders, as well as cancer and age related problems in the functioning of cells. And apart from helping in the treatment of these disorders, it could also be a very valuable experimental tool in the hands of biologists wishing to study mitochondria.