SARS-CoV-2: Know The Coronavirus Strain Behind The Devastating COVID19 Pandemic
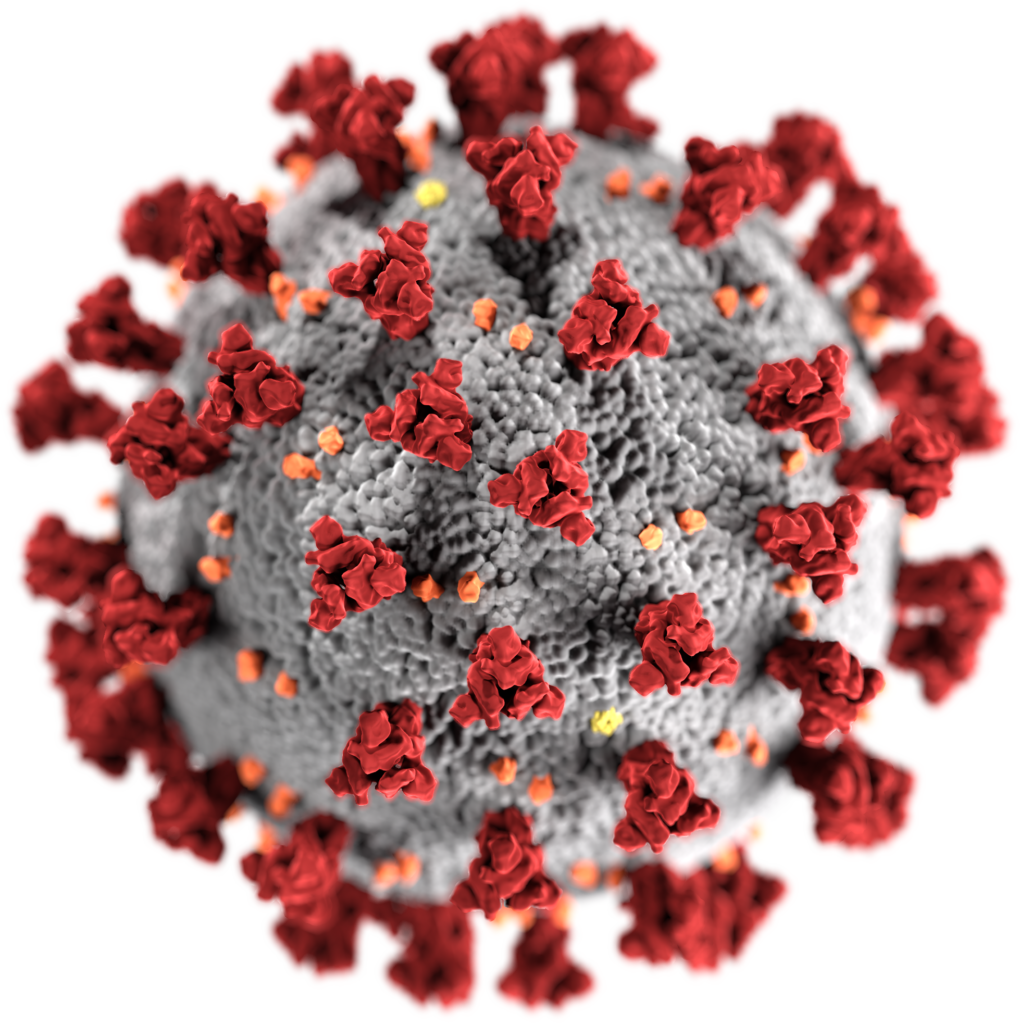
As the COVID19 pandemic ravages the world, its turning out to be one of the most devastating pandemics of our times. However, there have also been other outbreaks caused by coronavirus (the same virus behind COVID19) in the past. The 2002 SARS outbreak, which was also caused by a Coronavirus strain, killed just 774 people. The 2009 Swine flu outbreak (caused by an Influenza virus strain) killed 18,036 people people from January 2009 to August 2010. The current COVID19 outbreak on the other hand, has killed an astounding 266,000+ people world wide in just five months from January 2020 to April 2020, and as I write this, unfortunately, this figure is climbing every hour.
The culprit at the center of this whole thing is SARS-CoV-2, a newly encountered, previously unknown strain of coronavirus, that is closely related to SARS-CoV, the coronavirus strain responsible for the 2002 SARS outbreak. But what is it that makes SARS-CoV-2 so much deadlier than its 2002 cousin? In this post, we will discover the strengths and weaknesses of this new enemy in our midst, strengths and weaknesses that will hopefully inform the decisions of doctors, researchers and governments in dealing with the pandemic and helping the world come out of it.
Structure Of Coronavirus
Coronaviruses are typically 50-200 nm in diameter. To give you an idea of how tiny this is, the width of a human hair is around 80,000 nm. The outer shell of coronavirus, that you typically see in the images, is a hollow sphere made of lipid bilayer. This sphere, called viral envelope, is very similar to liposomes, which are being used for drug delivery in healthcare. You can read more about liposomes and drug delivery in this previous post by me. Both liposomes and viral envelope are made of lipid bilayer and have certain protein molecules embedded on their surface, which helps them target a unique type of cell in the body.
In fact, many viruses are so similar to liposomes that they are being engineered for use as drug delivery vehicles. Called virosomes, they are basically the outer envelopes of viruses that carry therapeutic drugs instead of the viral genes inside them. Consequently, they insert the drugs instead of the viral genes in the target cells. Virosomes can either be used to deliver conventional drugs to target cells, which can include cancer cells, or deliver antigens to immune system cells as a novel method of vaccination. Virosomes derived from influenza virus have been used so far, and virosomes based on HIV are being developed as an anti-HIV vaccine.
Coronaviruses have three different types of proteins on the surface of their envelope, namely the S (spike) protein, E (envelope) protein and the M (membrane) protein. Inside the sphere resides a long RNA molecule, which carries the genetic information of the virus. In other words, all of the virus’s genes reside in this RNA molecule, and each gene produces a unique protein that performs a unique function for the virus. This includes the genes for the S, E and M proteins found on the virus’s surface, as well as the gene for the N (nucleocapsid) protein, which covers and protects the RNA molecule itself.
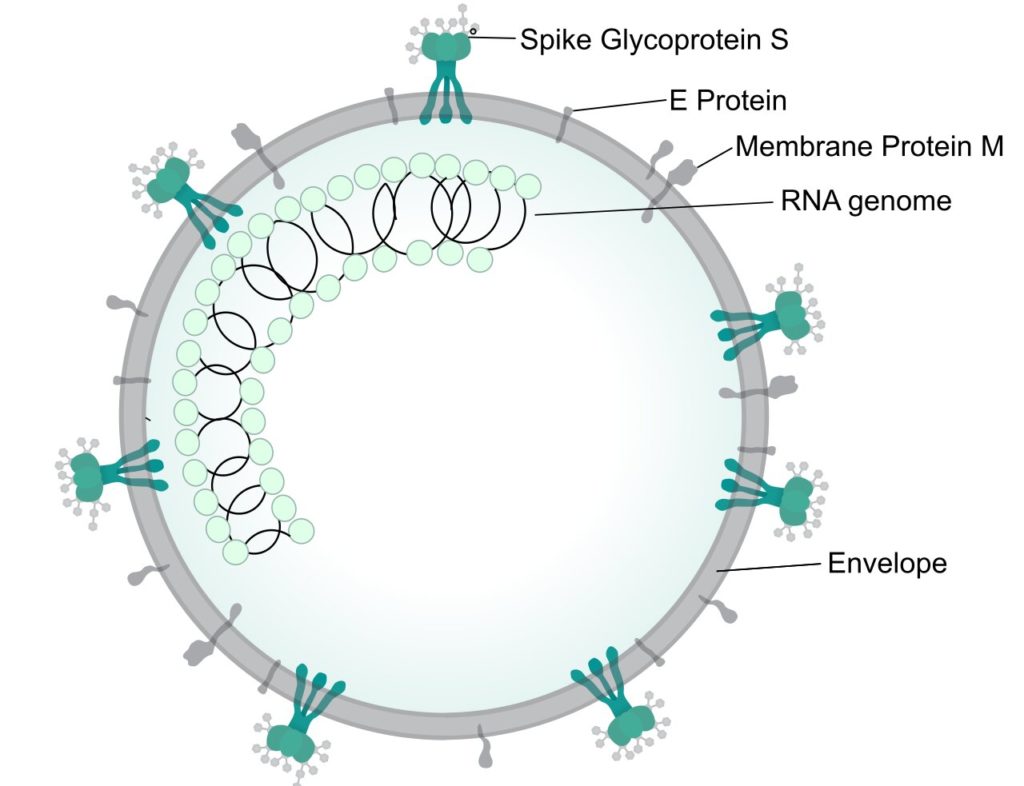
Chemically speaking, RNA is closely related to its more well known cousin, DNA. Most organisms, including humans, carry their genes in long DNA molecules. I have described the basic structure of DNA and how it stores genetic information in this previous post by me. A few viruses, including HIV and Coronaviruses, are the only organisms that use RNA instead of DNA to store genetic information. The most conspicuous difference between DNA and RNA is that DNA is double stranded (which is seen as two threads intertwined in the DNA structure we all know), while RNA is single stranded. Further details about RNA are beyond the scope of this post, but the figure below shows the differences between DNA and RNA.
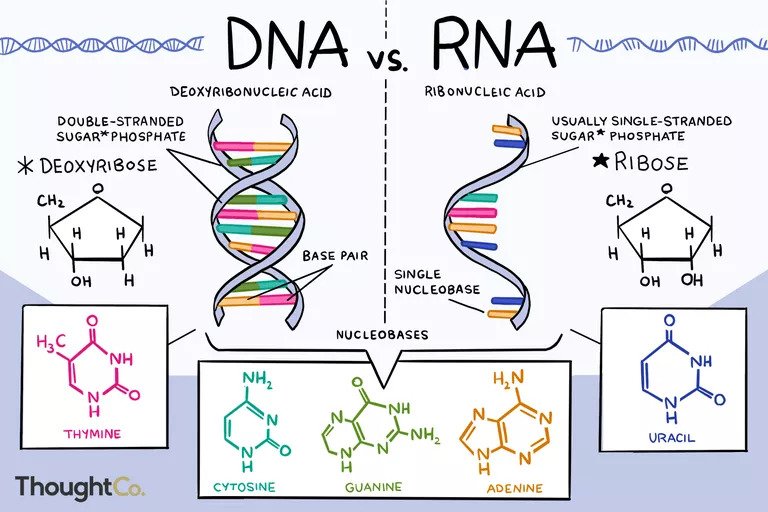
In the coming sections, we will see what the strengths and weaknesses of coronavirus are, and how researchers are trying to exploit the different vulnerabilities of the virus to deal with the current COVID19 pandemic.
Soap Disrupts The Outer Envelope Of Coronavirus
One thing whose role in absolutely transforming the health and life expectancy of humans because of how common it is now, is soap. Soap is single handedly responsible for saving millions of lives every year, which would otherwise be lost to severe infections. Not many realise that while its common practice today to wash your hands with soap daily, just a few centuries ago, even doctors wouldn’t wash their hands before performing surgeries. It was only in the late 18th century, that awareness about the role of hygiene in reducing rates of infection by pathogenic microbes led to wide spread use of soap. And this is why the authorities have been advocating washing your hands with soap to combat COVID19.
Coronavirus, just like most microbes, is vulnerable to soap because its envelop is composed of lipid bilayer, which is dissolved by soap molecules. This is because the bilayer is composed of phospholipid molecules that have a hydrophilic tip and a hydrophobic tail. Soap molecules too have a hydrophilic tip and a hydrophobic tail. As the saying goes, like dissolves like. The hydrophobic tails of the soap and phospholipid molecules interact with each other, while the hydrophilic tips of the soap molecules make it possible for the soap and phospholipid mixture to efficiently mix with water. This whole process of dissolving a hydrophobic substance in water using soap is called emulsification.
An Antibody Could Be Made Against The S Protein
One of the most important parts of the coronavirus from the standpoint of its ability to infect, is the S protein. This protein is composed of three identical subunits, and binds to Angiotensin Converting Enzyme 2 (ACE2). ACE2 is a protein found on the surface of many types of cells in the body, including the cells in the lungs, arteries, heart, kidney and intestines. This is why coronavirus can affect not just lungs but other organs of the body as well.
After the coronavirus binds to the ACE2 on a human cell using its S protein, the envelop of the virus fuses with the plasma membrane of the human cell, and the inner contents of the virus, namely the RNA and the N proteins, are released into the human cell. This fusion is possible because both the viral membrane and the plasma membrane of the host cell are similar in their chemical structure, with both consisting of a lipid bilayer. An alternative way in which coronavirus can enter the host cell after binding to ACE2 is endocytosis. Both these processes are shown in the video below.
Hence, one of the obvious targets for attacking the coronavirus is the S protein. And efforts to do that involve generating an antibody that binds to the S protein of the virus, and thus prevents it from binding it to the ACE2. Antibodies are produced by the body’s immune system when it is exposed to the foreign protein, which in this case, is the S protein of the coronavirus. Efforts are on in labs around the world to develop one or more antibodies against the S protein of coronavirus.
Two different approaches can be taken to do this. In one approach, researchers are trying to generate antibodies that bind to the S protein in other animals, which will then be administered to humans. This process, however, takes time. In the other approach, which is quicker, the plasma of people who have recovered from COVID19 is administered to others, as this plasma too would contain antibodies against the S protein, that the immune system of the person has generated on coming in contact with the virus. This approach is called convalescent plasma therapy and might be one of the quickest ways to deal with COVID19.
Chloroquine And Hydroxychloroquine Could Prevent Release Of Coronavirus From Endosome
As the video above shows, there is one more way, apart from membrane fusion, that a virus like SARS-CoV-2 can use to enter the human cell, and that is endocytosis. And this is where chloroquine and hydroxychloroquine, two antimalarial drugs that have been at the center of intense debate for their possible antiviral action, enter the scene. As their names suggest, chloroquine and hydroxychloroquine are very closely related in terms of their molecular structure. And both of them are believed to prevent the virus from gaining access to the interior of the cell by preventing its release from the endosome.
Lopinavir And Ritonavir Prevent Proteolysis Driven Activation Of Viral Proteins
Once the viral RNA is inside the host cell, it starts using the host cell’s protein synthesis machinery to produce the various viral proteins. These viral proteins need to be acted upon by certain proteases (enzymes that cleave proteins), to become functional. Two drugs, namely lopinavir and ritonavir, act on this step. They are protease inhibitors, preventing the protease enzymes from activating the viral proteins through proteolysis.
Remdesivir Prevents The Replication Of Viral RNA
Once the viral proteins are functional, some of them start the process of making copies of the viral RNA, to incorporate them in the new copies of the virus to be produced. One of these proteins is the RNA dependent RNA Polymerase. Remdesivir is a drug that prevents RNA dependent RNA polymerase from carrying out its function, and hence prevents the creation of new viral RNA copies.
Remdesivir was originally developed by Gilead Sciences to target Ebola virus, which is also an RNA virus just like SARS-CoV-2 and other coronaviruses. Subsequently it was also found to work against several other RNA viruses, like the MERS-CoV (Middle East respiratory syndrome-related coronavirus), and the original SARS-CoV responsible for the 2002 pandemic. It is therefore hoped that remdesivir would work against SARS-CoV-2 as well, and tests to determine this are currently ongoing. These drugs and treatment strategies I have listed are just the tip of the ice berg of different molecules and strategies that are currently being tested around the world to treat COVID19. The diagram below shows just how vast and complex the landscape of various strategies being tested is.
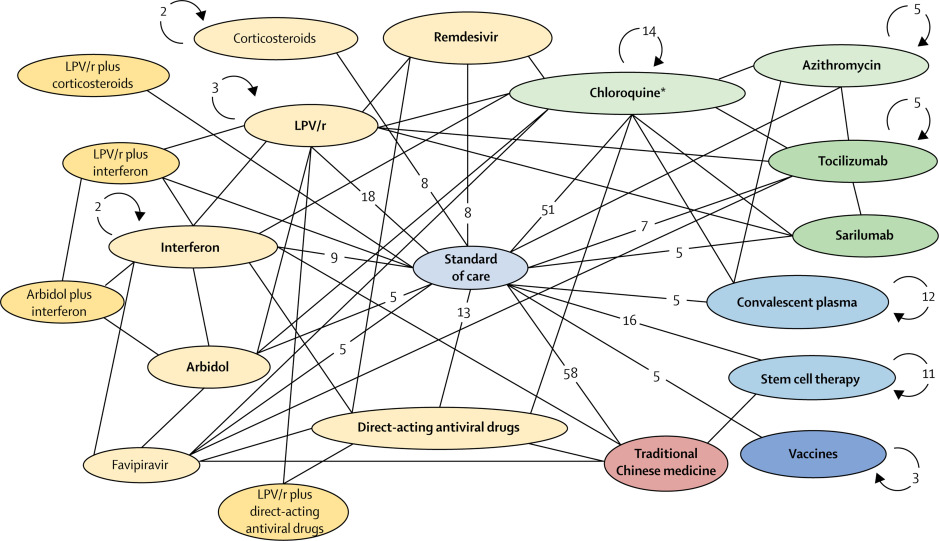
High Mutation Rate Could Be A Big Weapon In The Hands Of Coronavirus
One of the reasons why researchers haven’t been able to device a vaccine against many viruses so far, including HIV and Influenza viruses, is that these viruses have a very high rate of mutation. This means that the genetic information in their genes keeps undergoing subtle changes due to mutations. The frequency with which these viruses undergo mutation is much higher than higher organisms like humans. Theoretically, this higher rate of random mutations could result in many copies of the virus not being fit enough to survive.
In reality however, the sheer number of virus copies that are produced after infection of each host cell means that from time to time, a mutation will create a new version of the virus that, in the best scenario could render a vaccine useless, and in the worst case scenario, could be far more infectious and deadly than the original version of the virus. In fact, this is the reason why we suffer from flu almost every year. Because while our immune system now has antibodies against the influenza virus that infected us last year, it doesn’t have an antibody against the new mutant of influenza virus infecting us this year, making it play whack-a-mole with the constantly mutating virus.
Its also the reason why mutants of viruses deadlier than the previous mutants are regularly created, like the Avian flu and Swine flu mutants of influenza virus, the 2002 SARS-CoV mutant of coronavirus that is deadlier than original coronaviruses, and of course the SARS-CoV-2 mutant of coronavirus responsible for COVID19, that is even deadlier than the SARS-CoV we encountered in 2002. This high mutation rate is perhaps the biggest weapon in the hands of SARS-CoV-2 as well.
One of the major reasons why SARS-CoV-2 is so much more infectious than the original SARS-CoV of 2002 is that the S protein of the former binds to ACE2 far more strongly than the former. This too is the result of random mutations in the virus. Its amazing to contemplate that increased strength of binding between two tiny protein molecules, changed what could have been just another SARS outbreak like the one in 2002, killing just 774, into a global pandemic that has killed more than 266,000 around the world so far, with those figures climbing by the hour.
One way to deal with high mutation rate in coronavirus would be to use in treatment, not just one antibody, but a cocktail of different antibodies, each binding to a different part of the same molecule. For example, if two different antibodies bind to two different places on the S protein of coronavirus, then even if one of them is rendered useless due to a mutation in S protein that prevents the binding, the other antibody will still bind to S protein and prevent it from binding to ACE2. Because the likelihood of the binding sites for both antibodies on S protein mutating simultaneously and thus rendering both antibodies useless is extremely low.
Similarly, at least theoretically, cocktails of multiple drugs like lopinavir and remdesivir could be administered to increase chances of COVID19 being treated despite a mutation rendering one of the drugs useless. However, while antibodies are natural biomolecules and therefore rarely have side effects, drugs like lopinavir and remdesivir, being essentially foreign molecules, need to be evaluated for side effects and contraindications. Before a cocktail of such drugs is accepted as a viable mode of treatment, it must be ensured that the different drugs in the cocktail would not act in synergy to produce some serious side effect in the patient, that they don’t produce in isolation.
Conclusion
Teams of researchers around the world are racing to develop all kinds of therapies against COVID19, ranging from drugs, both newly discovered and old ones, to vaccines, monoclonal antibodies, and convalescent plasma therapy. I am sure many more molecules and strategies are currently under study for this purpose, than I have listed in this post, not to mention many different kinds of vaccines.
And eventually, we will be out of the woods just like in case of every other pandemic we have faced in the past. But I hope that the lessons learnt from this chapter make governments around the world fund medical research to accelerate its pace, so that when we face the next pandemic, we might be better prepared.
Nicely explained and a great read! very informative.
Thank you.